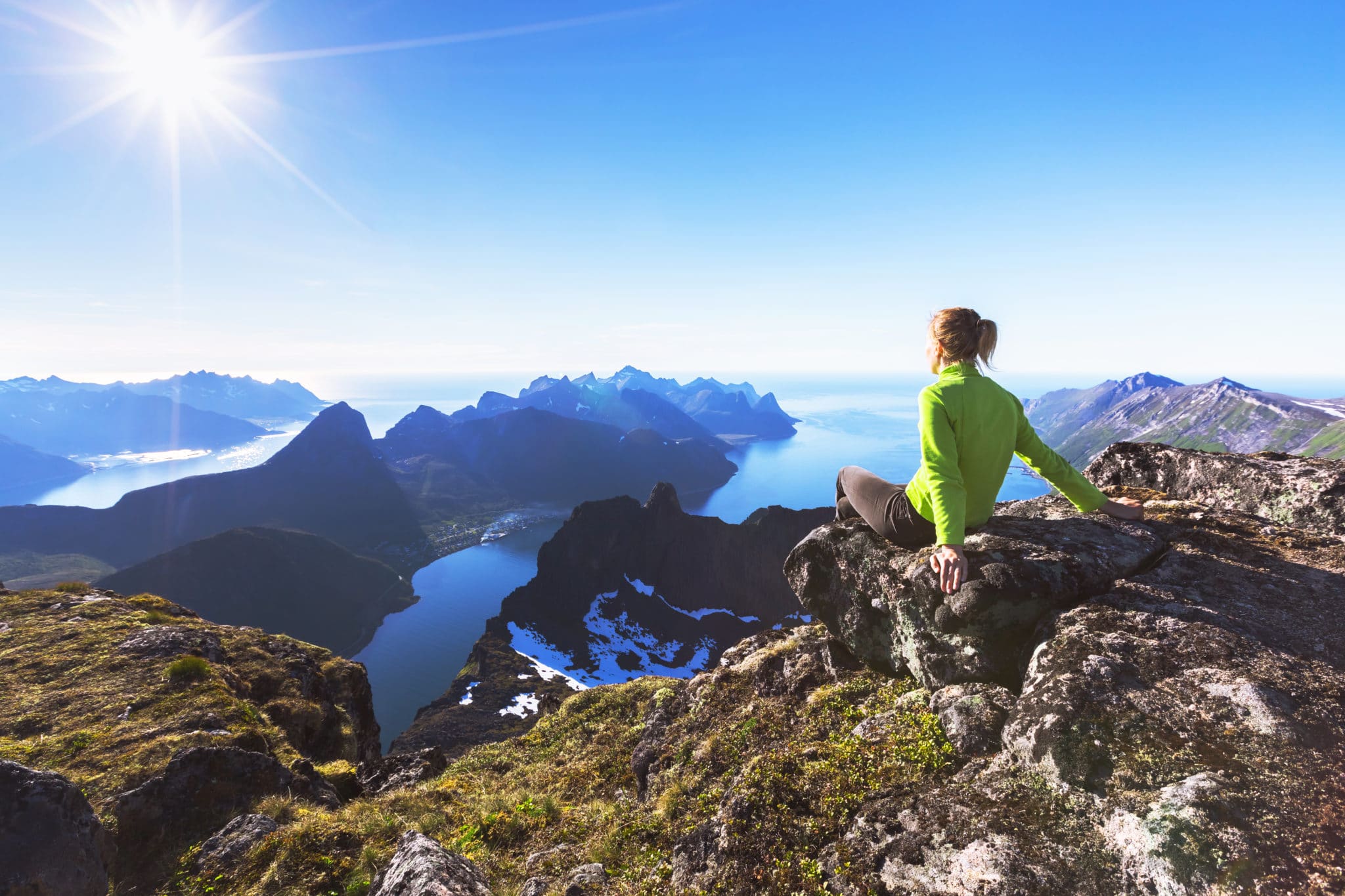
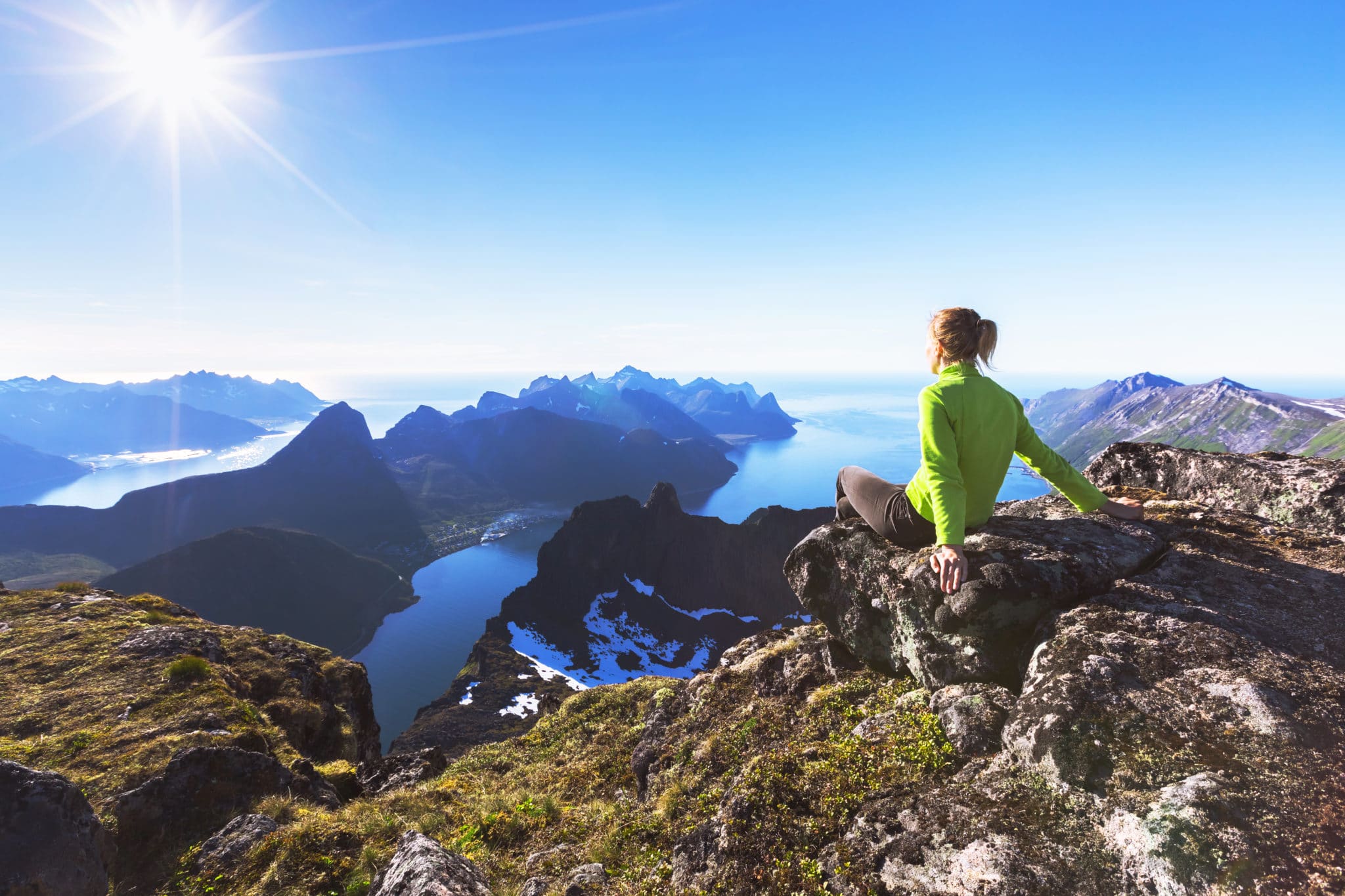
Human metabolism has evolved to be remarkably flexible in its ability to use a variety of dietary energy substrates. From a cultural history perspective, humans have demonstrated the ability to subsist for generations on up to 80% of dietary energy as carbohydrates at one end of the macronutrient spectrum to over 80% as fat (a ketogenic diet) at the other end. Given this wide range of dietary options, what is the optimum nutrient mix for humans? Is one end of this spectrum better than the other? Or is it best to be somewhere in the middle? If adopting one end of the spectrum, how long does human metabolism take to optimize its use of the dominant fuel provided? Surprisingly, the truthful answer to all of these is ‘we really don’t know!’ Yes, we know that a lot of disease symptoms and health indicators get much better soon after someone starts on a ketogenic diet. What we do not yet fully understand are all of the underlying physiological process through which ketosis promotes metabolic healing, and how long that process takes to get the full benefits.
Part of the reason that we don’t know more about this issue is the lack of longer-term human research at the high fat end of this dietary spectrum. Most people, including most nutrition scientists, appear to believe that the effects of a high fat diet can be determined after only a week or two. Thus most diet studies, particularly those assessing effects on physical performance, have typically been run for two weeks or less. And this is still the case, even though we published data over 3 decades ago showing that the process of adapting to a very low carbohydrate intake requires at least a month and likely quite a bit longer. How much longer? It depends upon what measure of adaptation one is following, and it is very likely that inter-individual variability is a factor as well.
While a ketogenic diet can put you into a state of nutritional ketosis in a matter of days, it can take weeks to months to become fully keto adapted.
One simple assumption that we can dispose of right away is that keto-adaptation occurs simultaneously with the build-up in the level of ‘ketones’ (primarily beta-hydroxybutyrate) in the blood. This hypothesis is based on the assumption that all of the benefits of ketones are directly linked to the amount available in the circulation. But here’s the catch – in our controlled inpatient studies, blood levels of BOHB come up to a new steady state within a week of starting a ketogenic diet (Phinney, 1980; Phinney, 1983), but one’s subjective and objective ability to do vigorous exercise takes anywhere from several weeks to a few months to recover and then stabilize. In other words, the process of keto-adaptation that allows for normal or increased exercise performance lags well behind the level of ketones in the blood.
Another indicator of how the body adapts to tight carbohydrate restriction may be the amount of glycogen that is maintained in muscles. Long ago, we noted that even when dietary carbohydrate is very low, muscle glycogen only drops to about half of its prior level after 4-6 weeks on a high fat diet (Phinney, 1980; Phinney, 1983). But more recently, we studied highly trained runners who had followed a ketogenic diet for 6 months or longer. Despite impressive weekly training mileage, their glycogen levels had come all the way back up to those of a matched group of athletes following a high carb diet (Volek 2016). This implies that the body’s ability to produce and defend muscle glycogen via gluconeogenesis can become finely tuned, but that this takes much longer than 4-6 weeks to occur.
An intriguing potential indicator of the body’s progress into keto-adaptation is the response of the serum uric acid content after initiation of a ketogenic diet. In healthy normal humans with initially normal blood uric acid levels, their values typically double in the first week of nutritional ketosis. Although this phenomenon was reported over half a century ago, its cause remains poorly understood. Some medical experts assume that carbohydrate restriction causes more purines from nucleic acids to break down to uric acid, either due to increased death of cells or from a higher dietary protein intake (i.e., meat containing nucleic acids). For reasons we won’t detail, neither of these mechanisms stands up to data in the published literature.
In short, the figure below depicts the serum uric acid levels typical for a healthy person fed a moderate protein ketogenic diet for 12 weeks. The acute rise in the first week occurs simultaneously with the increase in blood ketones, but then the slow progressive decline occurs despite stable levels of dietary protein and blood ketones. In other words, the initial rise in blood uric acid appears linked to the onset of nutritional ketosis, but then the body slowly adapts back to normal uric acid clearance despite sustained ketones in the blood. So what gives?
The best available answer to this question is that there is a somewhat murky understanding of how our kidneys deal with organic acids. To protect the body’s acid-base balance against too much acid from the diet or produced by our metabolism, our kidneys have the capacity to identify and actively clear organic acids from the blood. To some degree, at the onset of nutritional ketosis, this seems to be indiscriminate – it treats uric acid (which can be toxic when it builds up in the blood) and non-toxic levels of ketones all the same. So at the start of nutritional ketosis, these two organic acids compete for excretion, causing blood uric acid to rise despite no increase in its production. This was demonstrated long ago when two studies demonstrated sharp increases in blood uric acid levels when ketones were infused intravenously into human volunteers (Lecocq, 1965; Goldfinger, 1965).
But then, over time something seems to occur that slowly allows the body to efficiently rid itself of uric acid despite persistent blood ketones. In other words, the kidneys adapt to normalize uric acid excretion in the presence of beta-hydroxybutyrate, but for reasons we have yet to fathom, this process takes a few months to occur. One clue about how this occurs is the observation that during starvation ketosis, after 3 weeks the kidneys are excreting ketones at one third the rate than after just 4 days despite having the same blood ketone levels (Fery, 1985). This implies that over many weeks, the kidneys ‘learn how’ (i.e., adapt) to conserve ketones and get back to efficiently excreting uric acid.
In pointing out this slow recovery in the kidney’s handling of organic acids, we are not suggesting that this process itself is the limiting factor in keto-adaptation. Rather, perhaps other aspects of the body’s energy regulation and homeostasis are undergoing similar slow changes as well with the net effect resulting in the process we long ago named ‘keto-adaptation’ (Phinney, 1980; 1983).
Another potential structural change that might directly contribute to keto-adaptation would be an increase in mitochondrial density in muscle, brain, and other oxidative tissues. The dramatic shift in energy metabolism towards fatty acid and ketone oxidation would be expected to enhance mitochondrial function. Although human evidence of keto-adaptation being linked with mitochondrial adaptations are lacking (we should be in a position to change that soon), mouse studies indicate a connection (Bough et al. 2006; Ahola-Erkkila et al. 2010). This could occur by either increased mitochondrial biogenesis, or decreased mitochondrial damage and autophagy.
It is understood that reactive oxygen species (ROS) cause structural and functional damage to mitochondria, and that nutritional ketosis decreases mitochondrial ROS production. This could result in a prompt increase in the lifespan of existing mitochondria. Given estimates that mammalian mitochondria have half-lives in the 1-2 week range, and since it takes about 5 half-lives to reach equilibration after a change, an increase in mitochondrial lifetime might take 5-10 weeks to reach a new steady state for enhanced mitochondrial density, depending on the tissue or organ in question.
To be sure, when someone initiates a well-formulated ketogenic diet, a number of changes are set in motion which may occur in parallel, but with widely varying rates of completion. These may include:
With the increasing availability of oral ketone supplements, we should also be able to learn more about both the short-term effects of ketonemia and the selective effects of exogenous BOHB in contrast to the mix of BOHB and AcAc that is released by the liver (Owen 1969). Given the observation of Cahill et al. that AcAc is selectively taken up by muscle, then reduced to and re-secreted into the circulation as BOHB, there is the added potential benefit of this process in regenerating NAD+ in peripheral tissue. This offers a point of study for inter-organ fuel exchange along with a shift in cellular redox state between the liver and peripheral organs (e.g., muscle and potentially brain).
Bottom line: keto-adaptation will likely be defined as the net effect of many parallel responses to a well-formulated ketogenic diet, with these various responses occurring on differing timelines, and to differing degrees across individual phenotypes/genotypes. These many variables aside, however, the timeline for full keto-adaptation will likely be measured in months rather than days or weeks.
Have more questions about nutritional ketosis? Check out our FAQ by Dr. Steve Phinney and the Virta team.
The information we provide at virtahealth.com and blog.virtahealth.com is not medical advice, nor is it intended to replace a consultation with a medical professional. Please inform your physician of any changes you make to your diet or lifestyle and discuss these changes with them. If you have questions or concerns about any medical conditions you may have, please contact your physician.
1. Ahola-Erkkila S, Carroll CJ, Peltola-Mjosund K, Tulkki V, Mattila I, Seppanen-Laakso T, Oresic M, Tyynismaa H, Suomalainen A. Ketogenic diet slows down mitochondrial myopathy progression in mice. Hum Mol Genet, vol. 19, no. 10, pp. 1974-1984, 2010.2. Bough KJ, Wetherington J, Hassel B, Pare JF, Gawryluk JW, Greene JG, Shaw R, Smith Y, Geiger JD, Dingledine RJ. Mitochondrial biogenesis in the anticonvulsant mechanism of the ketogenic diet," Ann Neurol, vol. 60, no. 2, pp. 223-235, 2006.3. Fery F, Balasse FO. Ketone body production and disposal in diabetic ketosis. Diabetes. 1985; 34:326-332.4. Forsythe C, Phinney SD, Feinman RD, Volk BM, Freidenreich D, Quann E, Ballard K, Puglisi MJ, Kraemer WJ, Maresh CM, Bibus D, Fernandes ML, Volek JS. Limited effect of dietary saturated fat on plasma saturated fat in the context of a low carbohydrate diet. Lipids. 2010; 45:947-952.5. Fox IH, Halperin ML, Goldstein MB, Marliss EB. Renal excretion of uric acid during prolonged fasting. Metabolism Volume 25, Issue 5, May 1976, Pages 551-5596. Goldfinger S, Klinenberg JR, Seegmiller EJ, Miller J, Bradley K. Renal Retention of Uric Acid Induced by Infusion of Beta-Hydroxybutyrate and Acetoacetate. N Engl J Med. 1965; 272:351-3557. Kim DY, Hao J, Liu R, Turner G, Shi F-D, Rho JM. Inflammation-Mediated Memory Dysfunction and Effects of a Ketogenic Diet in a Murine Model of Multiple Sclerosis. PLoS ONE 2012; 7:e35476.8. Lecocq FR, McPhaul JJ Jr. The effects of starvation, high fat diets, and ketone infusions on uric acid balance. Metabolism. 1965; 14:186-1979. McSwiney FT, Wardrop B, Hyde PN, Lafountain RF, Volek JS, Doyle L. Keto-adaptation enhances exercise performance and body composition responses to training in endurance athletes. Metabolism. 2017; in press.10. Owen OE, Felig P, Morgan AP, Wahren J, Cahill GF Jr. Liver and Kidney Metabolism during Prolonged Starvation. J Clin Invest. 1969; 48:574-58311. Owen OE, Reichard GA Jr. Human forearm metabolism during progressive starvation. J Clin Invest 1971; 50:1536-1545.12. Phinney SD, Horton Es, Sims EAH et al. The capacity for moderate exercise in obese subjects after adaptation to a hypocaloric ketogenic diet. J Clin Invest. 1980 66;11-52-1161.13. Phinney SD, Bistrian BR, Wolfe RR, Blackbiurn GL. The human metabolic response to chronic ketosis without caloric restriction: physical and biochemical adaptation. Metabolism 32:757-768.14. Volek JS, Freidenreich DJ, Saenz C, Kunces LJ, Creighton BC, Bartley JM, Davitt PM, Munoz CX, Anderson JM, Maresh CM, Lee EC, Schuenke MD, Aerni G, Kraemer WJ, Phinney SD. Metabolic characteristics of keto-adapted ultra-endurance runners. Metabolism. 2016; 65:100-1015. Youm YH, Nguyen KY, Grant RW, Goldberg EL, Bodogai M, Kim D, D'Agostino D, Planavsky N, Lupfer C, Kanneganti TD, Kang S, Horvath TL, Fahmy TM, Crawford PA, Biragyn A, Alnemri E, Dixit VD. The ketone metabolite β-hydroxybutyrate blocks NLRP3 inflammasome-mediated inflammatory disease. Nat Med. 2015; 21:263-9